Cell Division Cycle Group
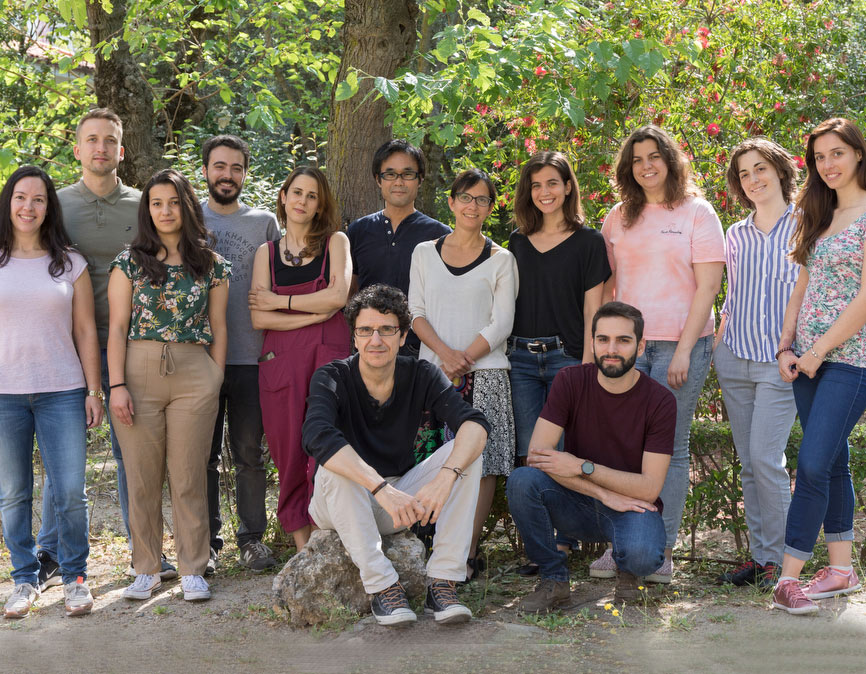
Research Lines
Cell cycle deregulation is a common feature of human cancers and multiple therapeutic strategies are aimed to inhibit the cell division cycle in tumor cells. Current efforts can be broadly divided into three different groups:
- a) preventing the commitment to cell cycle entry imposed by oncogenic signals.
- b) abrogation of the cell cycle checkpoints to increase lethality in highly proliferating cells.
- c) taking advantage of the special sensitivity of cells to abnormal chromosome segregation during mitosis.
The two first approaches are clearly based on the specific signals originated from the oncogenic stress in tumor cells. This has been far more difficult to achieve in targeted therapies directed against mitosis given the conservation in the essential mechanism that regulates chromosome segregation in normal or tumor cells.
Our group is interested in understanding the physiological relevance of major cell cycle regulators, and studying the possible use of inhibitors against major cell cycle engines for cancer therapy.
Our laboratory is interested in addressing some specific questions with relevance in normal physiology and malignant transformation.
Which are the major links between cell cycle progression and metabolic regulation?
Are these processes controlled by cell cycle kinases and phosphatases?
Does metabolic reprogramming have consequences in cell proliferation?
Do cancer cells coordinately change their cell cycle and metabolic consequences?
How major regulatory pathways such as RAS- or PI3K-dependent routes coordinate cell cycle progression with metabolic changes?
Is this control specifically regulated during the different cell cycle phases?
Which of these mechanisms can be used for cancer therapy?
Terminal differentiation is usually coupled to permanent exit from the cell cycle. The expression levels of many cell cycle regulators typically decline when cells exit the cell cycle and undergo differentiation. Moreover, induction of the expression of anti-proliferative molecules during cell differentiation prevents activation of major cell cycle engines such as cyclin–CDK complexes in terminally differentiated cells. This effect is mostly promoted by two types of molecules: a) enzymes involved in the proteasome-dependent degradation of cell cycle proteins; and b) protein phosphatases that counteract the function of cell cycle kinases. These enzymes dot not only modulate cell cycle progression but are critical players in the balance between cell proliferation and differentiation, a balance of major implications during development, tissue homeostasis or disease.
During the chromosome cycle, the chromatin undergoes multiple changes required for the control of transcription, but also higher-level reorganizations required for the proper segregation of sister chromatids during cell division. The epigenetics code, based on a variety of post-translational modifications in histones, is critical for both of these processes. Mitotic kinases, such as Aurora B or Haspin are known to phosphorylate histones to promote some of the changes required for cell cycle progression.
We are currently investigating the regulatory crosslinks that may control chromatin structure during cell cycle progression, or differentiation/undifferentiation, including the changes required for reprogramming to pluripotent cells or the differentiation of these cells towards multiple tissue types.
We have identified a microRNA -previously described as an oncosuppressor- that is preferentially expressed in the 2C-morula stages during preimplantation development in the mouse embryo. By using a variety of in vitro and in vivo approaches, we have reported that transient exposure of already-established induced pluripotent stem cells (iPSC) or ESCs to this miRNA promotes naive pluripotency improving the ability of these PSCs to differentiate into multiple cell lineages and to reach further maturation properties without interfering with their self-renewal capacity.
Transient expression of the miRNA in PSCs leads to greater developmental potential in chimeric or tetraploid complementation assays. In addition, human iPSCs exposed to this miRNA generate interspecies human-mouse conceptuses with higher efficiency than control cells.
Mechanistically, these effects are mediated through the miRNA-dependent control of de novo DNA methyltransferases Dnmt3a and Dnmt3b, thereby regulating the DNA methylation landscape of these miRNA-treated PSCs. These observations suggest that the developmental and differentiation potential of already established PSCs can be readily enhanced by transient exposure to a single microRNA.
Reprogramming technology offers the potential to treat many diseases, including neurodegenerative diseases, cardiovascular disease, and amyotrophic lateral sclerosis (ALS). In theory, easily-accessible cell types (such as skin fibroblasts) could be biopsied from a patient and reprogrammed, effectively recapitulating the patient’s disease in a culture dish.